Feature
Ulrich Steidl, M.D., Ph.D.: A Potential New Approach to Treating Blood Cancers
December 3, 2024
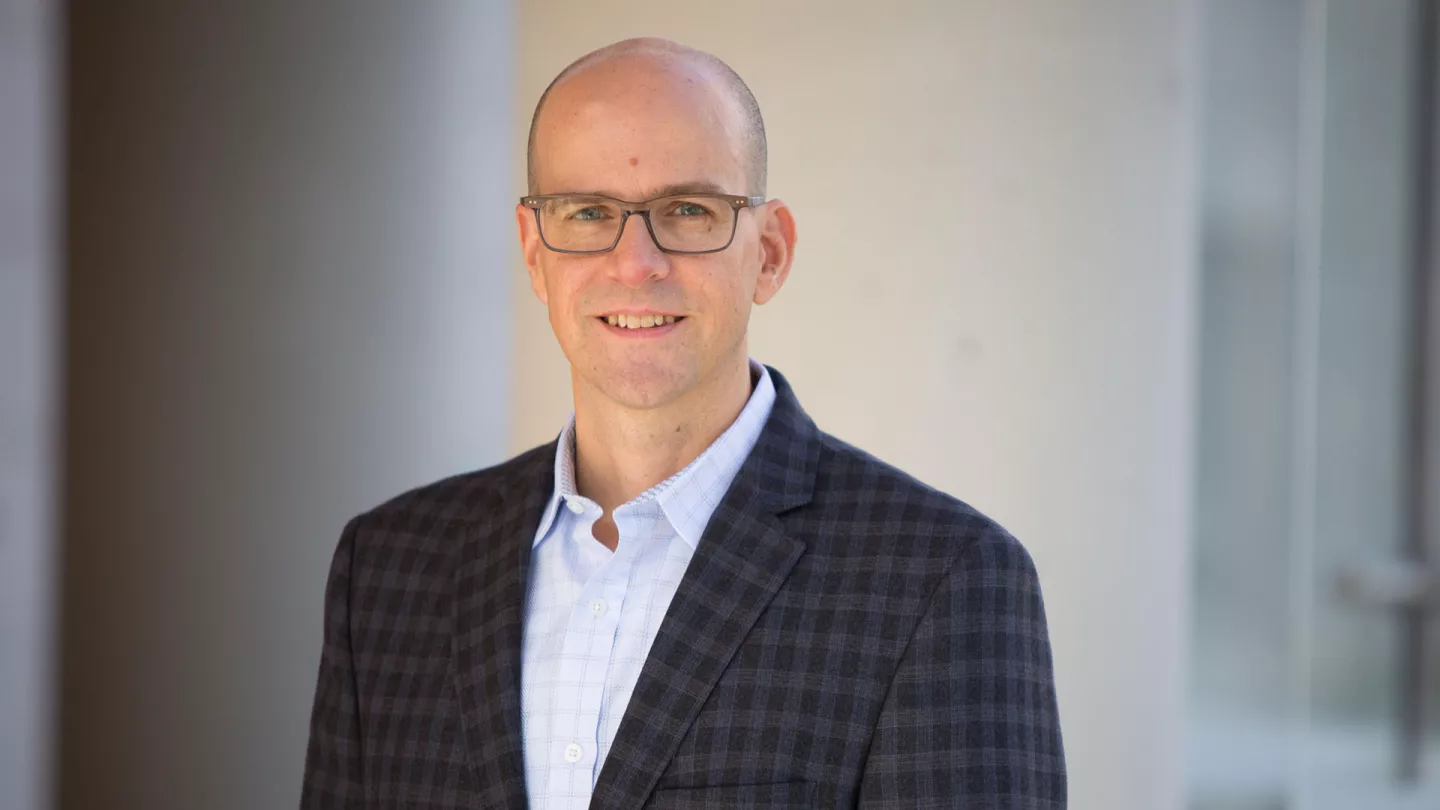
Genes attract a lot of attention in discussions about health and disease. But transcription factors (TFs)—the proteins that turn genes on and off—are the genome’s real movers and shakers. Cancer can result when TFs act abnormally, but researchers haven’t found a way to successfully influence TFs—until now.
Einstein and Montefiore researchers have developed a strategy for “redistributing” TFs, pointing to a possible approach for treating acute myeloid leukemia (AML) and other blood cancers. Their findings were described this past September in Nature Genetics.
The paper’s corresponding author, Ulrich Steidl, M.D., Ph.D., is professor and chair of cell biology and the Edward P. Evans Endowed Professor for Myelodysplastic Syndromes at Albert Einstein College of Medicine, deputy director of the National Cancer Institute-designated Montefiore Einstein Comprehensive Cancer Center (MECCC), and interim director of the Ruth L. and David S. Gottesman Institute for Stem Cell Research and Regenerative Medicine. Lead author Samuel Taylor, Ph.D., is a staff scientist in cell biology and a member of Dr. Steidl’s research team.
Below, Dr. Steidl explains his team’s new strategy regarding TFs and how it advances Einstein and Montefiore’s efforts to improve treatments for deadly blood cancers.
Explain why your lab focuses on AML.
AML is one of the most challenging blood cancers to treat. Our lab and others at MECCC and our stem cell institute have discovered key drivers of AML and other cancers that arise from abnormal stem cells, which are extremely difficult to eradicate. Only about 30% of patients with AML survive past five years, and Einstein and Montefiore researchers and clinicians are working to develop therapies to improve survival. Our new study presents a promising new avenue to treat AML and other blood cancers, which all have genetic origins.
Before we get to your study, tell us more about TFs.
The genome is essentially the same in every cell in our bodies, but our cells are extremely different. These differences are due to TFs. They act collectively, and the complex interactions among different TFs are referred to as transcription “networks.” These networks are responsible for deciding which genes are “turned on,” or expressed, and which are “turned off,” or repressed. So, they really determine the fate of a cell, for good or ill. In cancer and other diseases, TF networks are often corrupted.
How do TFs know where to go?
TFs recognize and bind to specific DNA sequences, which is how they influence the expression of nearby genes. TFs interact with chromatin, the complex of DNA and proteins that make up chromosomes in cells. Chromatin essentially condenses the long strands of DNA into a more compact shape. And chromatin is dynamic; its structure is constantly changing, which influences whether TFs can gain access to the DNA of genes to turn them on or off.
How do errors in TF contribute to disease?
The TF known as PU.1 is a prime example. PU.1 plays a major role in controlling how blood cells develop. It regulates an array of genes that tell blood-forming stem cells to differentiate into different types of mature blood cells—the white blood cells, red blood cells, or platelets that the body needs. PU.1 is impaired in many types of AML. Defective PU.1 causes blood-forming stem cells to become stuck in an intermediate, nonfunctional state, leading to fewer mature blood cells and the proliferation of immature, malignant blood cells.
Can abnormal TF activity be corrected?
It has previously proved extremely difficult to interfere with TFs in a targeted manner. One major problem is that TFs often lack stable, well-defined binding sites that drugs can easily target.
Your new study suggests a wholly new approach to correcting PU.1 abnormalities. How did you discover it?
We showed in a 2017 study that when we used small-molecule drugs to block the sites on DNA where PU.1 binds, we decreased the number of leukemic cells and increased survival in an animal model of AML. In our new study, we conducted experiments involving novel versions of these PU.1 DNA-binding drugs and AML stem cells to learn more about what was happening at the molecular and cellular levels.
Because PU.1 molecules were unable to dock at their primary DNA binding sites, they became redistributed to other parts of the genome. This repositioning of PU.1 to “alternate” target sites caused two things to happen. First, chromatin became altered in a way that profoundly changed which genes were accessible to TFs. Second, in a stunning discovery, we observed that the repositioned PU.1 activated genes that made AML stem cells differentiate towards mature blood cells—in effect “curing” AML, at least at the cellular level.
Essentially, we’ve uncovered a biological phenomenon that we call “pharmacologically induced TF redistribution,” which could have far-reaching implications. We hope our findings will motivate researchers to investigate other disease-related TFs and the effects of redistributing them using small-molecule drugs. If this strategy can be harnessed and used clinically, it could eventually lead to an entirely new approach for cancers and other conditions driven by dysfunctional TFs.
What comes next in this line of research?
We’re now working to develop even more potent TF-redistributing compounds and evaluate their therapeutic potential in AML, myelodysplastic syndromes, and bone marrow failure. I want to emphasize that we’re a long way from making TF-redistributing drugs available to patients, but we see this work as a promising start.
More information:
Dr. Steidl’s paper is “Pharmacological restriction of genomic binding sites redirects PU.1 pioneer transcription factor activity.” Additional Einstein authors include Amit Verma, Ph.D., interim chair of the department of oncology and professor of oncology, of medicine, and of developmental & molecular biology, Mendel Goldfinger, M.D., associate professor of oncology and of medicine, and Kristy Stengel, Ph.D., assistant professor of cell biology. The paper and a companion research briefing were published online September 18 in Nature Genetics.
Albert Einstein College of Medicine has intellectual property related to this research and is seeking licensing partners able to further develop and commercialize this technology. Interested parties can contact the Office of Biotechnology and Business Development at biotech@einsteinmed.edu.