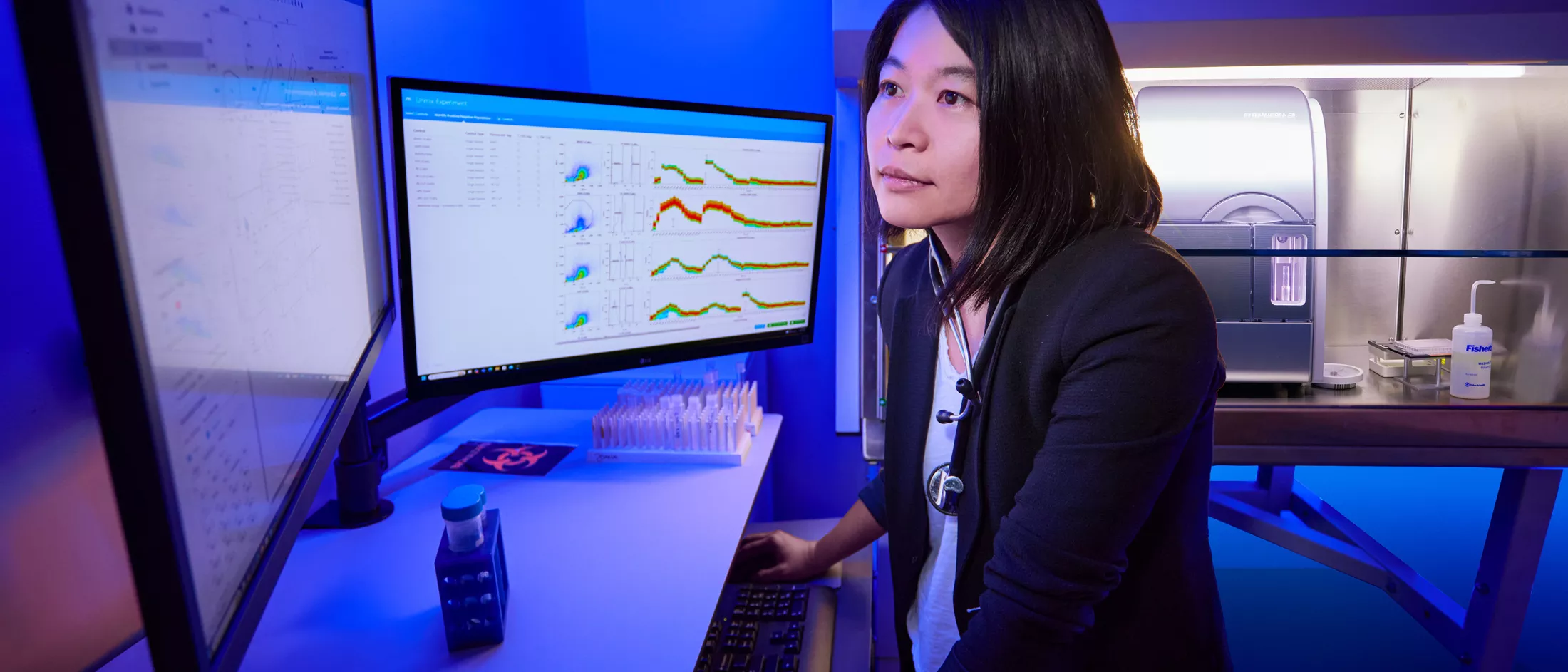
Science at the Heart of Medicine
The New York Center for Rare Diseases at Montefiore Einstein is at the forefront of innovation and rare disease research, committed to finding new ways to predict, prevent, diagnose and treat rare conditions. As a National Organization for Rare Disorders (NORD)-designated Rare Disease Center of Excellence, we offer a research-focused, evidence-based approach to care. Our world-renowned physician-scientists and scientists collaborate closely across disciplines and with investigators worldwide, leading research and spearheading numerous studies and clinical trials. This gives our patients access to emerging technologies and innovative treatments and cures that may otherwise be unavailable.
Our research teams are actively conducting basic, translational and clinical research to explore the genetic mechanisms, natural history, epigenomics and population genetics of rare diseases and develop diagnostic and therapeutic solutions for a broad range of rare conditions. From co-developing the clinical guidelines setting the standard of care for acid sphingomyelinase deficiency to spearheading the nation’s largest pilot newborn rare diseases screening study (ScreenPlus), we continue to lead the way in rare disease research. We are committed to serving children and adults who are underrepresented in rare disease research and ensuring that a diverse population of patients is represented in our clinical trials. We have also partnered with GeneDx, PacBio and the Genomics team in Google Research to help identify the genetic causes and best treatments for rare diseases that have eluded diagnosis in diverse communities.
Our basic science and translational research programs are focused on numerous compelling areas of rare disease research at the cellular and molecular levels. From investigating the mechanisms of human disease, transcriptional regulation and DNA sequence variation in the non-coding majority of the genome, and why rare diseases occur in an individual or family, to exploring the mechanisms responsible for birth defects in 22q11.2 deletion syndrome (22q11.2DS) and neurodegeneration in RNA polymerase III-related leukodystrophy, and developing gene therapy for hereditary red blood cell disorders, our researchers are pushing the boundaries of modern science.
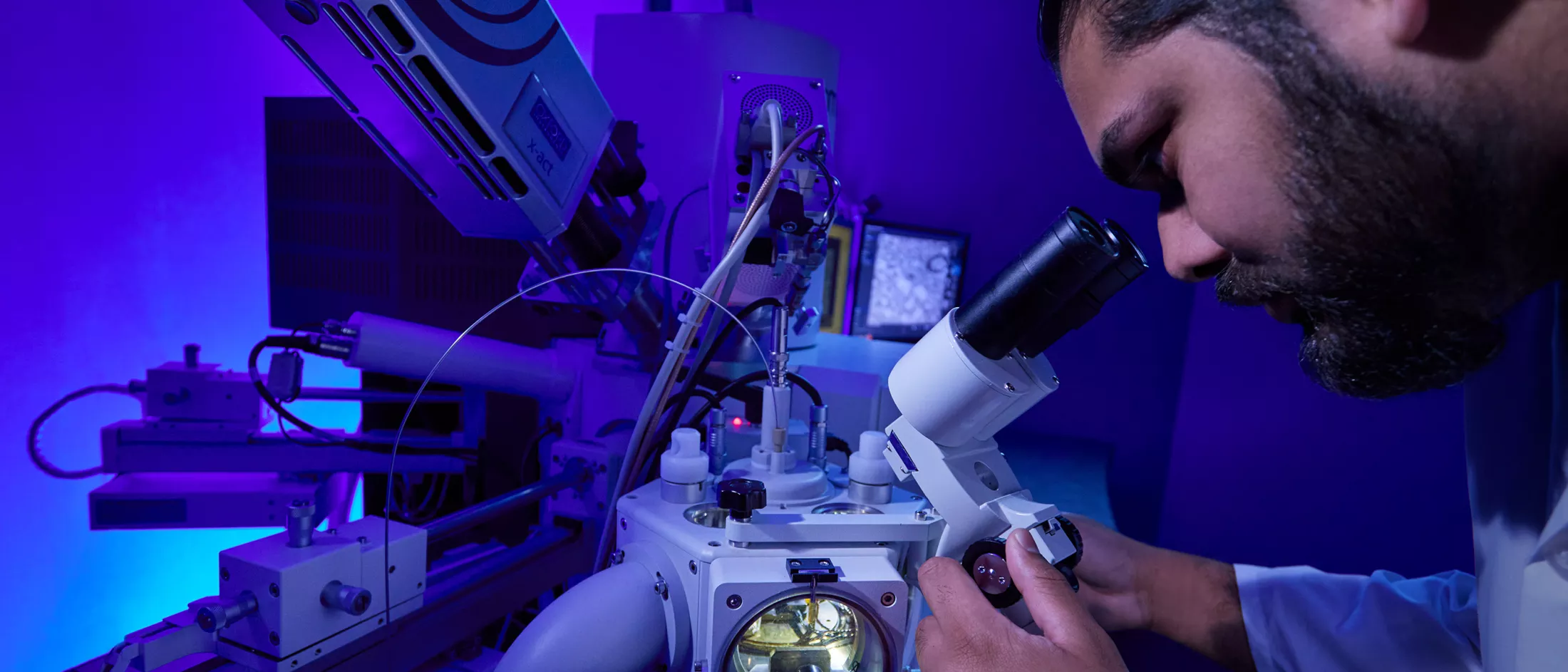
Basic & Translational Research Institutes and Initiatives
The New York Center for Rare Diseases at Montefiore Einstein is dedicated to advancing rare disease research and translating scientific breakthroughs into groundbreaking diagnostics and treatments. Explore our comprehensive suite of research laboratories, institutes and initiatives.
Mutations in the SAMHD1 gene can cause a rare genetic disorder called Aicardi-Goutières syndrome (AGS), which leads to chronic activation of the immune system and inflammation. Individuals with AGS suffer from progressive neurological damage, resulting in cognitive decline and, ultimately, death. The Diaz-Griffero lab is using human primary cells from AGS patients, as well as a mouse model, to investigate the role of SAMHD1 in the disease.
Recently, the Diaz-Griffero lab discovered that mitochondrial DNA in AGS patients triggers the activation of a protein called cGAS, which leads to the chronic immune activation seen in the disease. Based on these findings, the lab is now testing drugs that inhibit cGAS, aiming to find compounds that reduce or prevent this harmful immune response in patients. To advance potential treatments for AGS, the lab plans to screen several compound libraries to identify novel inhibitors that could be directly used to treat the disorder. With support from the Intellectual and Developmental Disabilities Research Center (IDDRC), the Diaz-Griffero lab is making rapid progress toward identifying molecules that could be effective in treating AGS.
The X-linked form of Charcot-Marie-Tooth disease (CMT1X), due to mutations in Connexin32, is the second most prevalent form of hereditary motor and sensory neuropathy, characterized by progressive muscle atrophy and weakness, areflexia and variable sensory abnormalities. Using a mouse CMT1X model, the Stanley lab, in collaboration with others, has shown that the secreted proteoglycan isoform of colony-stimulating factor-1 (pgCSF-1) is involved in the pathogenic inflammation of this peripheral nervous system disease. They showed successful targeting of the pgCSF-1 isoform and not the membrane-spanning cell surface or secreted glycoprotein isoforms to be desirable as a therapeutic approach.
Their goal is to develop humanized monoclonal antibodies to human pgCSF-1. To this end, they have teamed up with Dr. Jonathan Lai of the Department of Biochemistry at Albert Einstein College of Medicine to develop antibodies that selectively target this isoform. These antibodies can be tested in a humanized CSF-1 Connexin32 mouse model. They are currently testing methods to assess the effectiveness of the high-affinity anti-spgCSF-1 antibodies so far developed in the humanized mouse model.
The Secombe lab uses the vinegar fly Drosophila and human induced pluripotent stem cells (iPSCs) to model Claes-Jensen syndrome. This neurodevelopmental disorder is characterized by altered cognition, including intellectual disability, epilepsy, autism spectrum disorder, sleep disturbances and aggressive tendencies. Claes-Jensen syndrome is caused by pathogenic variants in the KDM5C gene, which encodes a chromatin-modifying enzyme that regulates the expression of many genes within the genome.
The Secombe lab aims to define how KDM5C regulates gene expression programs within the brain to understand the causes of the symptoms associated with Claes-Jensen syndrome and identify potential avenues for therapeutics. They combine state-of-the-art multi-omics approaches to assess the effects of specific patient-associated variants on gene expression and neuronal morphology, and to correlate this with altered cognition, epilepsy and sleep, all of which can be modeled in Drosophila. They also use human iPSC-induced neurons to understand how KDM5C functions in the brain’s different cell types, such as excitatory and inhibitory neurons. They work closely with families with Claes-Jensen syndrome through the KDM5C Awareness Research Education and Support (KARES) Foundation. Dr. Julie Secombe is Chair of the KARES Foundation Scientific Advisory Board.
The Kalpana lab has accumulated a wealth of knowledge about how SMARCB1 functions and its structure-function relationships. By looking at SMARCB1-associated mutations in Coffin-Siris syndrome, they investigate the mechanism by which these mutations affect SMARCB1 function in chromatin remodeling and transcription and cellular function. Their goal is to investigate the global effect of these mutations on chromatin remodeling and transcriptional regulation. Specifically, they aim to identify specific gene and pathway defects that could be targeted therapeutically, similar to their previous success in identifying drugs that target specific SMARCB1 and Integrase domains to treat HIV-1 infection. By identifying drugs that target either the mutated regions of SMARCB1 in Coffin-Siris syndrome or the altered downstream effectors, they hope to develop effective treatments for the syndrome.
Human congenital aniridia (OMIM#106210) is a rare panocular disease (prevalence ~1:72,000) defined by foveal hypoplasia and malformation, and/or absence of the iris together with other ocular abnormalities, such as cataracts, corneal dystrophies, glaucoma and nystagmus.
These abnormalities are caused by heterozygous mutations in the PAX6 gene (chromosome 11p13, OMIM# 607108) encoding a sequence-specific DNA-binding transcription factor. PAX6 is often called the “master” regulatory gene of eye development. As its model system, the Cvekl Lab uses retinal and lens organoids generated from induced pluripotent stem cells (iPSCs) carrying various PAX6 heterozygous and homozygous mutations.
The Stanley lab has developed a mouse model of CSF-1 receptor (CSF1R) related leukoencephalopathy (CRL) that faithfully reproduces the symptoms and pathology of the human disease, an adult-onset dementia caused by dominant inactivating mutations of the CSF1R in which approximately 90% of cases have been misdiagnosed as Alzheimer’s disease. Using the mouse model, they have confirmed that CRL is a primary microgliopathy and, as such, is also relevant to several other neurodegenerative diseases in which microglia play prominent roles, including ALS, Alzheimer’s disease and multiple sclerosis. They have identified two novel targets for the prophylactic treatment of CRL mutation carriers and developed a prophylactic treatment for the disease that prevents symptom development and pathology in the mouse model.
Their goal is to understand how CSF1R mutations in microglia cause neuronal death and deleterious effects on other neural cell types, including oligodendrocytes. They have used single nuclear RNAseq to identify the other cell types involved and coupled this with proteomics to identify the proteins and pathways affected. They are currently investigating how particular proteins are involved in disease development to identify other therapeutic approaches.
The Morrow lab takes human and mouse genetic approaches to understand the cause of birth defects associated with DiGeorge syndrome/chromosome 22q11.2 deletion syndrome (22q11.2DS). Approximately 60% of patients have congenital heart disease, among other medical problems. Studies of rare disorders with known causes, like 22q11.2DS deletion, can identify genes that are responsible for more commonly occurring birth defects in the general population. TBX1 encoding a transcription factor and CRKL encoding a cytoplasmic signaling protein are two of the most important genes on 22q11.2. Their goal is to identify altered downstream genes and genetic pathways in mouse models of 22q11.2DS. They work closely with Drs. Deyou Zheng and Zhengdong Zhang at Albert Einstein College of Medicine.
As part of the lab’s human genetic studies, they performed whole genome sequencing to identify genetic modifiers, in part with patients recruited at the Montefiore Einstein Regional Center for 22q11.2 Deletion Syndrome. They are currently analyzing this data to understand why some patients with the same size hemizygous deletion on 22q11.2 are severely affected, while others are only mildly affected. Their data indicate that genetic modifiers of 22q11.2DS can serve as risk factors for congenital heart disease in the general population. Once they understand the molecular mechanisms, it will be possible to develop novel therapeutics to improve patient care.
The Gennerich lab employs advanced biochemistry and single-molecule microscopy to study the molecular mechanisms of cytoplasmic dynein, a microtubule-associated motor protein responsible for retrograde cargo transport. Mutations in dynein and its cofactor proteins, Lis1 and NudE, lead to neurodevelopmental and neurodegenerative disorders, collectively known as dyneinopathies, which include spinal muscular atrophy (SMA), SMA with lower extremity predominance (SMALED), malformation of cortical development (MCD) and other related conditions. These diseases are poorly understood, partly due to the unclear functions of dynein’s accessory proteins. For example, while Lis1 and NudE mutations cause lissencephaly and microcephaly, respectively, how these cofactors regulate dynein function remains unclear. The Gennerich lab combines single-molecule fluorescence and optical tweezers-based force measurements with innovative protein engineering to investigate how dynein, in complex with its activator dynactin and cargo adaptors, is regulated by Lis1 and NudE. These studies aim to provide new insights into the molecular basis of dynein-linked diseases, potentially identifying targets for therapeutic intervention.
In collaboration with Dr. Melissa Wasserstein, Chief of the Division of Pediatric Genetic Medicine at Montefiore Einstein, the Gennerich lab is characterizing the effects of disease-causing mutations in cytoplasmic dynein at both the patient and molecular levels. Their research seeks to unravel the molecular causes of these associated disorders, with the ultimate goal of developing therapies. By pioneering novel single-molecule techniques and leveraging advanced molecular tools, such as CRISPR/Cas9 and unnatural amino acids, the Gennerich lab is advancing the study of cytoplasmic dynein, providing critical insights into motor protein function in both health and disease.
The Meier lab focuses on understanding the molecular causes of dyskeratosis congenita (DC), a rare bone marrow failure syndrome. Patients with DC struggle to produce enough blood cells to transport oxygen and fight infections. The most common cause of DC is mutations in proteins that interact with a variety of small noncoding RNAs, which are essential for many cellular functions. They identified and cloned the cDNA for the protein most often mutated in DC, called NAP57 (now also known as dyskerin), which is encoded by the DKC1 gene. While one function of these RNA-protein complexes, telomere extension, is known to be impaired in DC patients, it is unclear whether other functions are also disrupted. They aim to determine how different mutations in NAP57 affect its pseudouridine synthase activity, which is guided by small H/ACA RNAs. To do this, they are collaborating with two labs that have the country’s largest collections of DC patients. Their research seeks to discover new treatment options for DC and potentially other bone marrow failure syndromes.
The Kurshan lab uses the roundworm C. elegans to model and characterize patient mutations in the gene CACNA1A, which encodes a voltage-gated calcium channel involved in synaptic transmission, and mutations that lead to diverse symptoms and syndromes, including episodic ataxia, hemiplegic migraine, epilepsy, and spinocerebellar ataxia.
Their goal is to understand why these mutations lead to pleiotropic patient symptoms. In collaboration with the Intellectual and Developmental Disabilities Research Center (IDDRC), they have been characterizing a mutation initially identified in a patient at Montefiore Einstein. They found that this mutation leads to different effects in excitatory versus inhibitory neurons. Using CRISPR, they are generating and characterizing additional transgenic worm models for variants of unknown significance. Finally, using forward genetic screens, they have identified additional calcium-signaling proteins that mediate these effects, suggesting potential alternative therapeutic avenues.
Germline RUNX1 mutation causes a familial platelet disorder and predisposes individuals to the development of myeloid malignancy at an early age of onset. The RUNX1 gene encodes a sequence-specific transcription factor that is critical for normal blood development. The Stengel lab seeks to better understand how RUNX1 haploinsufficiency alters platelet function and predisposes individuals to myeloid malignancy. Using cutting-edge, targeted protein degradation technology in mouse and cell-based models, coupled with multi-omics approaches, they are defining the mechanism(s) of RUNX1 transcriptional control. These mechanistic studies aim to improve our understanding of RUNX1-FPDMM disease etiology and to identify molecularly targeted therapies that may thwart the progression of FPD to malignancy.
The Steidl lab uses mouse models and human samples to model and characterize the effects of patient germline mutations in the gene RUNX1, which encodes a transcription factor and can lead to a spectrum of diseases called familial platelet disorder with associated myeloid malignancy (FPDMM).
Their goal is to understand why these mutations lead to a varying spectrum of hematologic symptoms, including thrombocytopenia, and why and how they can progress to myeloid malignancies, including myelodysplastic syndromes (MDS) and acute myeloid leukemia (AML), in some individuals (the lifetime risk is ~50%) but not in others. They have developed novel mouse models to study this progression to malignancies longitudinally. Interestingly, these novel models show many molecular and cellular commonalities with primary patient samples, including at the level of disease-initiating pre-leukemic and leukemia stem cells. The goal is to identify causative factors and triggers of the malignant transformation and to use this knowledge to test approaches to antagonize or delay this process preemptively. These efforts may lead to novel therapeutic avenues of precision prevention in this rare disease and resultant cancers.
The LMNA gene encodes for an intermediate filament protein that serves as a structural framework for the cell’s nucleus. This protein also plays an important role in gene silencing by sequestering heterochromatin close to the nuclear periphery. A single point mutation in the LMNA gene results in the production of a truncated version of the Lamin A protein (Progerin), causing abnormalities in the nuclear structure, and is the cause of the advanced aging disease Progeria.
Progerin is also made as part of normal aging, certain types of cancer and cellular senescence. The San Martin lab seeks to understand the impact of progerin accumulation on the three-dimensional folding of the genome within the nucleus. As stem cells differentiate, they require competent nuclear lamina to silence genes that are not required for their final function. The lab has characterized a progerin-dependent effect on early bone development and tissue repair that can result in stem cell depletion. This effect potentially affects downstream joint, fat, and vasculature formation in HGPS patients.
The lab uses fertilized chicken eggs as hosts for patient-derived cells to test cell differentiation in humanized in-vivo systems. They have identified metabolic interventions that partially rescue the stem cell identity and are characterizing changes in the genome architecture that mediate bone formation in these cells. Understanding how these genome folding dynamics are affected by the presence of progerin could reveal new therapeutic targets aimed at replenishing the stem cell niche.
The Lachman lab uses induced pluripotent stem cell (iPSC) technology to model several neurodevelopmental and neuropsychiatric disorders. One is Jansen de Vries syndrome (JdVS), which is characterized by a learning disability, severe anxiety, restricted eating and gastrointestinal problems. JdVS is caused by mutations in the terminal exons of PPM1D, which codes for a Ser/Thr phosphatase that acts as a negative regulator of p53 and other components of the DNA damage response (DDR) pathway (e.g., H2AX). JdVS-associated mutations are truncating (tr) nonsense and frameshifts that result in the removal of an N-terminal degradation signal that prolongs the half-life of the catalytic domain, which reduces the phosphorylation of p53 and other DDR proteins, thereby impairing DNA repair. Somatic truncating mutations are oncogenic; germline mutations cause JdVS (future cancer risk is unknown). For molecular and functional studies, patient and CRISPR-engineered iPSC lines are differentiated into glutamatergic neurons, cerebral organoids and microglia. A small subgroup of JdVS patients have acute-onset neuropsychiatric decompensation and regression that is also seen in Down syndrome and autism spectrum disorders. Along with PPM1D truncating mutations, the Lachman lab has found pathogenic mutations in several other DDR genes in children with this problem. The hypothesis is that defective DDR leads to the accumulation of cytosolic DNA in microglia and/or peripheral immune cells, which triggers an innate immune response and neuroinflammation.
Recessive mutations in CRB1 (OMIM #604210) cause retinal dystrophies, with early-onset Leber congenital amaurosis 8 as the most severe case, in which the patients are born blind. Currently, there is no cure for CRB1-associated retinal dystrophies. The Liu lab investigates how CRB1 mutations affect the human retina using human retinal organoids carrying CRB1 disease mutations. Retina organoids are “mini retina” and enable functional studies of retinal disease genes directly in human retinal tissue. The results of their studies will lead to therapeutic designs for preventing and delaying CRB1-associated disease progression in the human retina. As some CRB1-associated retinal dystrophies, such as retinitis pigmentosa 12, start during early childhood, therapeutic windows exist for these patients, and the benefit is life-changing. Dr. Liu has received NIH and private foundation grant support to study CRB1 and is a three-time winner of the 3D-ROC competition organized by the National Eye Institute, NIH.
Lowe syndrome (LS) is a rare X-linked disorder characterized by intellectual and developmental disability, congenital cataracts and renal proximal tubule dysfunction. It is caused by hypomorphic mutations in OCRL, which codes for inositol polyphosphate 5-phosphatase, a key enzyme involved in endosome recycling, clathrin-coated pit formation and actin polymerization. The Lachman lab has generated patient-specific and CRISPR-engineered induced pluripotent stem cells (iPSC) to produce glutamatergic neurons and neural progenitor cells (NPCs) to study LS, an especially critical model system because knockout (KO) mice do not have a behavioral phenotype. This could be due to a compensatory pathway that rescues mice from the effects of OCRL deficiency. An analysis of F-actin and the WAVE-1 complex revealed a marked disruption in both LS compared with control NPCs . A defect in early endosome recycling was also detected. The lack of a behavioral phenotype in KO mice suggests that hypomorphic OCRL variants found in most patients are more damaging than a complete KO because of a dominant-negative effect on compensatory pathways, as seen in mice. This is supported by the finding that knocking out the expression of a patient-specific hypomorphic OCRL variant using CRISPR-Cas9 editing rescued the F-actin and WAVE-1 abnormalities seen in LS NPCs. These studies show the value of iPSC-based disease modeling and present a unique treatment strategy for LS.
The Philadelphia-negative myeloproliferative neoplasms (MPNs) are rare blood cancers with limited treatment options. These include primary myelofibrosis (PMF; incidence of 0.33-0.46 cases per 100,000), polycythemia vera (PV; incidence of 1 in 36,000-100,000) and essential thrombocythemia (ET; incidence of 1-2.5 cases per 100,000). Both PV and ET can progress to PMF, and all three diseases can progress to acute myeloid leukemia. PMF has the poorest prognosis of the three MPNs, with a median survival of five to six years, and is characterized by the abnormal proliferation of hematopoietic stem cells, leading to the replacement of bone marrow with fibrous scar tissue (fibrosis). This fibrosis disrupts normal blood cell production, causing symptoms that impact patient quality of life, including severe cytopenias, splenomegaly and constitutional symptoms. The underlying pathophysiology of these MPNs involves mutations in genes such as JAK2, CALR and MPL, leading to increased JAK-STAT signaling, which promotes excessive cell proliferation and the release of pro-fibrotic cytokines, contributing to marrow fibrosis. Stem cell transplantation is the only curative treatment for PMF, but many patients are not eligible due to advanced age or comorbidities. While JAK inhibitors can improve spleen size and constitutional symptoms, they have minimal effects on bone marrow fibrosis and are not curative. Therefore, it is important to identify new therapeutic targets for MPNs, particularly for PMF.
The Gritsman lab recently published that the FDA-approved kinase inhibitor crizotinib can inhibit the proliferation of MPN cells in mouse models of PV (PMID 36537918) and can reduce JAK/STAT signaling. They identified RON kinase as the most important target of crizotinib in MPN cells and found that RON can physically interact with JAK2 to potentiate JAK/STAT signaling. Their current efforts are to develop new therapeutic approaches to target RON in MPN cells. RON is a potential cell surface target for antibody-mediated or cellular therapy approaches. They are currently using flow cytometry with anti-RON antibodies and single-cell multi-omics approaches with the Mission Bio Tapestri targeted DNA sequencing platform to determine whether MPN cells have elevated RON cell surface expression compared to healthy blood cells, and to correlate RON cell surface expression with the presence of MPN mutations. This will determine whether RON could be a safe cell surface target and whether RON should be studied as a biomarker of disease progression. They are also testing the genetic deletion of the RON cell surface domain or Tyrosine kinase domain in mouse models of PV and PMF to determine which of these domains plays the most important role in disease progression and bone marrow fibrosis. These studies will also inform future drug discovery efforts for more potent and selective RON kinase inhibitors or PROTACS to target RON in MPN patients.
Neurodevelopmental disorder with regression, abnormal movements, loss of speech and seizures (NEDAMSS) is a pediatric onset disease characterized by neurodevelopmental regression that becomes apparent usually before seven years of age, after normal early development in most patients. Gradual loss of language and motor skills (walking, feeding, facial weakness) and variable types of seizures are observed. The disease is currently incurable and the cellular and molecular mechanisms are poorly characterized. The impact of four NEDAMSS patient mutations has been studied by others in neurons and astrocytes derived from induced pluripotential stem cell-derived cells. The results point to a critical role of IRF2BPL in maintaining the ability of astrocytes to support neuronal survival.
The Stanley lab aims to establish a mouse IRF2BPL model of NEDAMSS. The site of the conditional IRF2BPL mutation was chosen based on the characterization of several human mutations occurring in the same region, all of which showed dominant negative properties by sequestering wild-type IRF2BPL in the cytosol. The mouse model will be validated using behavioral testing of motor function, complemented by endpoint histological and molecular characterization. Once validated, the model will permit investigation of the mechanisms underlying the development of NEDAMSS and the identification and evaluation of potential therapeutic agents. Because IRF2BPL physically interacts with IRF2BP2, a suppressor of type I interferon signaling, it is planned to explore the effects of mutation on microglia and the contribution of inflammation to NEDAMSS.
The Gennerich and Sosa labs combine cutting-edge molecular biology, single-molecule biophysics and cryoEM to investigate the molecular mechanisms of KIF1A, a neuron-specific motor protein crucial for nuclear migration in differentiating brain stem cells and the transport of synaptic precursors and dense core vesicles to axon terminals. Over 170 mutations, primarily in the motor domain, have been linked to KIF1A-associated neurological disorders (KAND), which include progressive spastic paraplegias, microcephaly, encephalopathies, intellectual disabilities, autism, peripheral neuropathy, optic nerve atrophy, and cerebral and cerebellar atrophy.
In collaboration with Wendy Chung, MD, PhD, Chief at Boston Children’s Hospital at Harvard Medical School, the Gennerich and Sosa labs are characterizing the molecular impact of disease-causing KIF1A mutations. Their research aims to unravel the molecular basis of KAND and solve cryoEM structures of mutant proteins, with the long-term goal of facilitating structure-based therapeutic development. By pioneering novel single-molecule techniques and utilizing advanced molecular tools like CRISPR/Cas9 and unnatural amino acids to express and study KIF1A, the labs are advancing the understanding of motor protein function in both health and disease.
The Greally lab studies the 98% of our DNA that does not encode genes. Functionally, this non-coding DNA contains the sequences that regulate how our genes are used. While it is now clear that variants in these regulatory loci can cause human genetic diseases, it has been very difficult to understand how to identify the single change that causes a patient’s rare disease among the millions of non-coding variants.
The lab uses advanced analytical approaches and molecular assays to identify the small subset of functional non-coding variants (fNCVs) in the genomes of patients with rare diseases. A current focus is on individuals with autism and intellectual disability, finding that an increased burden of de novo variants at regulatory loci of glutamatergic neurons is associated with lower IQ. The goal is to improve genomic diagnostics in individuals with neurodevelopmental disorders, as a necessary foundation for therapeutics.
The group uses patient cells and rodent models to study N-Glycanase 1 (NGLY1) deficiency, an ultra-rare, autosomal recessive genetic disorder caused by loss of function variants in NGLY1 a conserved protein that cleaves N-glycans from proteins. NGLY1 is the only cytosolic enzyme that catalyzes hydrolysis of the amide bond between an N-linked glycan and asparagine in proteins. Patients with NGLY1 deficiency display a range of multi-organ symptoms, including global developmental delay and/or intellectual disability, hyperkinetic movement disorder, hypo- or alacrima, and peripheral neuropathy. Loss of NGLY1 function has been known to affect cellular processes such as proteasomal homeostasis, signaling pathways and ferroptosis.
Their goal is to evaluate the impact of NGLY1 deficiency on autophagy and the lysosomal system, a crucial component of cellular proteostasis. Autophagy, a cellular recycling pathway, degrades macromolecules, protein aggregates and organelles in lysosomes. In collaboration with the Grace Science Foundation and the Intellectual and Developmental Disabilities Research Center (IDDRC) at Montefiore Einstein, they have found that NGLY1 deficiency leads to reduced autophagy activity and severe defects in lysosome function. They propose that lysosomal malfunctioning is responsible to a large extent for the loss of proteostasis and accumulation of aggregates of misfolded glycoproteins in this disease. They are currently using chemical modulators of autophagy/lysosomes to reduce cellular proteotoxicity with therapeutic purposes in NGLY1 deficiency.
The Willis lab has developed a series of mouse models to study a neurodegenerative disease caused by mutations in RNA polymerase (Pol) III subunits. This enzyme synthesizes essential non-coding RNAs found in all eukaryotic cells, yet biallelic mutations of its subunits cause a neurologic disorder, with patients diagnosed between birth and adolescence. The disease is characterized by a loss of motor function, cognitive deficits, hypo-myelination and cerebellar atrophy, and various non-neurologic features (hypodontia, myopia and hormonal abnormalities).
The research seeks to understand the cellular and molecular basis of disease pathogenesis. Current findings point to a causal role for a global reduction in transfer RNA in disease initiation and reveal differential sensitivities of different brain regions to a pathogenic Polr3a mutation. Next-generation sequencing technologies are being used to discover how changes in the Pol III transcriptome affect protein synthesis and the proteome. In addition, pre-clinical studies in mice are investigating genetic and pharmacological strategies to suppress disease progression and provide therapeutic options for patients.
The Jo lab uses the mouse model to investigate how the Magel2 gene influences energy metabolism and mental health, as this gene is implicated in the development of Prader-Willi syndrome (PWS). PWS, a neurogenetic disorder, results from the absence of paternal expression of a gene cluster in the human 15q11-q13 region. One of the hallmark symptoms of PWS is an unrelenting desire for food, which leads to a swift weight increase during the early years of life. Additionally, key phenotypic PWS behaviors include temper outbursts, anxiety, obsessive-compulsive behaviors and social cognition deficits. We developed a knockdown mouse model to examine how the loss of function of the Magel2 gene affects both energy balance and mental health. In this model, the Magel2 gene is deleted exclusively in appetite-suppressing proopiomelanocortin (POMC) neurons within the hypothalamus that innervate the medial amygdala, an area critical for emotional responses. This study helps establish a solid foundation for developing improved strategies to address both obesity and emotional disorders in individuals with PWS.
ScreenPlus is a consented, multi-disorder pilot newborn screening program implemented in conjunction with the New York State Newborn Screening Program (NBS). It gives families the option to have their newborn screened for a panel of additional conditions. The study has three primary objectives: 1) define the analytic and clinical validity of multi-tiered screening assays for a flexible panel of disorders, 2) determine disease incidence in an ethnically diverse population and 3) assess the impact of early diagnosis on health outcomes. Over a five-year period, ScreenPlus will screen approximately 100,000 infants born in eight high-birth-rate, ethnically diverse pilot hospitals in New York for a flexible panel of rare genetic disorders. This study will also evaluate the ethical, legal and social issues pertaining to NBS for complex disorders, which will be done via online surveys of ScreenPlus parents who opt to participate and through qualitative interviews with families of infants who are identified through ScreenPlus.
Thrombotic thrombocytopenic purpura (TTP) is caused by a deficiency in ADAMTS13, which can be either congenital or autoimmune in origin. Without ADAMTS13, von Willebrand factor (vWF) multimers are not properly cleaved, leading to severe microthrombosis associated with high mortality. Current treatments include plasma exchange or recombinant ADAMTS13 injections. However, for autoimmune forms of TTP, recombinant ADAMTS13 is ineffective due to inhibition by autoantibodies. We are developing human-engineered therapeutic cultured red blood cells that express antibody-resistant fragments of ADAMTS13 on their surface. In a mouse model, these cells have shown potential as a treatment for TTP.
Clinical Trials
We are spearheading all phases and types of clinical trials, some of which are sponsored by the National Institutes of Health (NIH), for individuals with rare diseases. By enrolling in a clinical trial, you can help our scientists develop new treatments, diagnostic tools and other strategies to prevent, diagnose and treat rare diseases. Through our clinical trials, you may also be able to access emerging technologies and innovative treatments that may otherwise not be available to the general public.
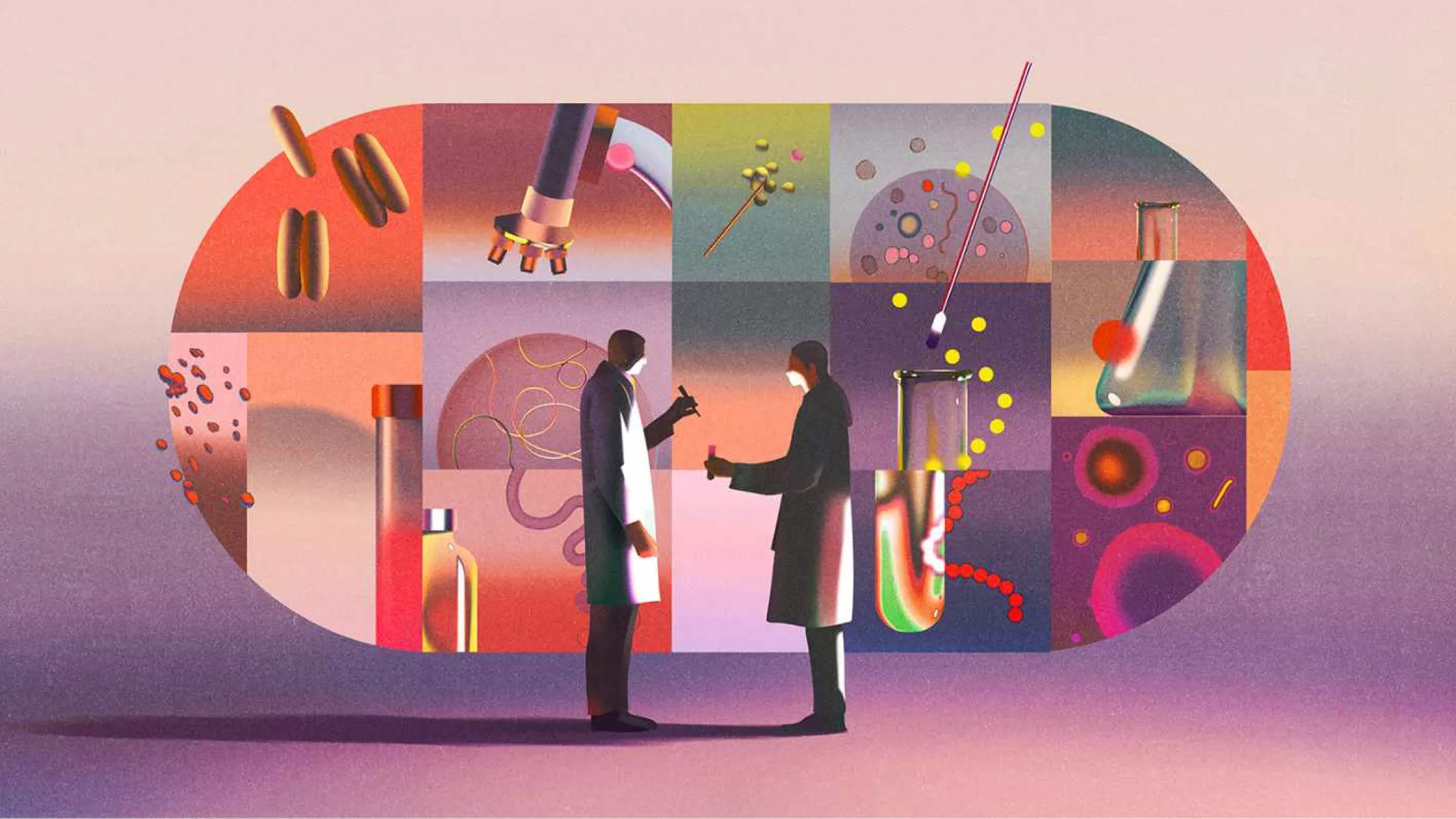
Featured Rare Disease Clinical Trials
Rett Syndrome Registry (RSR)
-
Start Date
August 2, 2022
-
Conditions
Rett Syndrome, Rett Syndrome (Atypical) , Genetic Disease, Genetic Diseases (X-Linked), Intellectual Disability, Neurobehavioral Manifestations, Neurologic Manifestations, Neurologic Disorder, Neurodevelopmental Disorders, Nervous System Diseases
ScreenPlus: A Comprehensive, Flexible, Multi-disorder Newborn Screening Program (ScreenPlus)
-
Start Date
May 10, 2021
-
Conditions
Acid Sphingomyelinase Deficiency, Ceroid Lipofuscinosis (Neuronal 2), Cerebrotendinous Xanthomatosis, Fabry Disease, GM1 Gangliosidosis, Gaucher Disease, Lysosomal Acid Lipase Deficiency, Metachromatic Leukodystrophy, Mucopolysaccharidosis II, Mucopolysaccharidosis III-B, Mucopolysaccharidosis IV A, Mucopolysaccharidosis VI, Mucopolysaccharidosis VII, Niemann-Pick Disease (Type C)
Lysosomal Acid Lipase (LAL) Deficiency Registry (ALX-LALD-501)
-
Start Date
May 30, 2013
-
Conditions
Lysosomal Acid Lipase Deficiency, Cholesterol Ester Storage Disease, Wolman Disease, Acid Cholesteryl Ester Hydrolase Deficiency (Type 2), LIPA Deficiency, LAL-Deficiency
Bronx Transthyretin Amyloid Cardiomyopathy Database
-
Start Date
September 23, 2023
-
Conditions
Transthyretin Cardiac Amyloidosis, Transthyretin Amyloid Cardiomyopathy
A Study Evaluating Gene Therapy with BB305 Lentiviral Vector in Sickle Cell Disease
-
Start Date
February 14, 2020
-
Conditions
Sickle Cell Disease
ATHN Transcends: A Natural History Study of Non-Neoplastic Hematologic Disorders
-
Start Date
September 30, 2020
-
Conditions
Hematologic Disorder, Bleeding Disorder, Connective Tissue Disorder, Hemophilia, Thrombosis, Von Willebrand Diseases, Thrombophilia, Rare Bleeding Disorder, Platelet Disorder, Factor IX Deficiency, Factor VIII Deficiency, Thalassemia, Sickle Cell Disease
MEK Inhibitor Mirdametinib (PD-0325901) in Patients with Neurofibromatosis Type 1 Associated Plexiform Neurofibromas (ReNeu)
-
Start Date
September 29, 2019
-
Conditions
Plexiform Neurofibroma, Neurofibromatosis Type 1 (NF1)
Nephrotic Syndrome Study Network (NEPTUNE)
-
Start Date
April 1, 2010
-
Conditions
Minimal Change Disease (MCD), Membranous Nephropathy, Glomerulosclerosis (Focal Segmental)
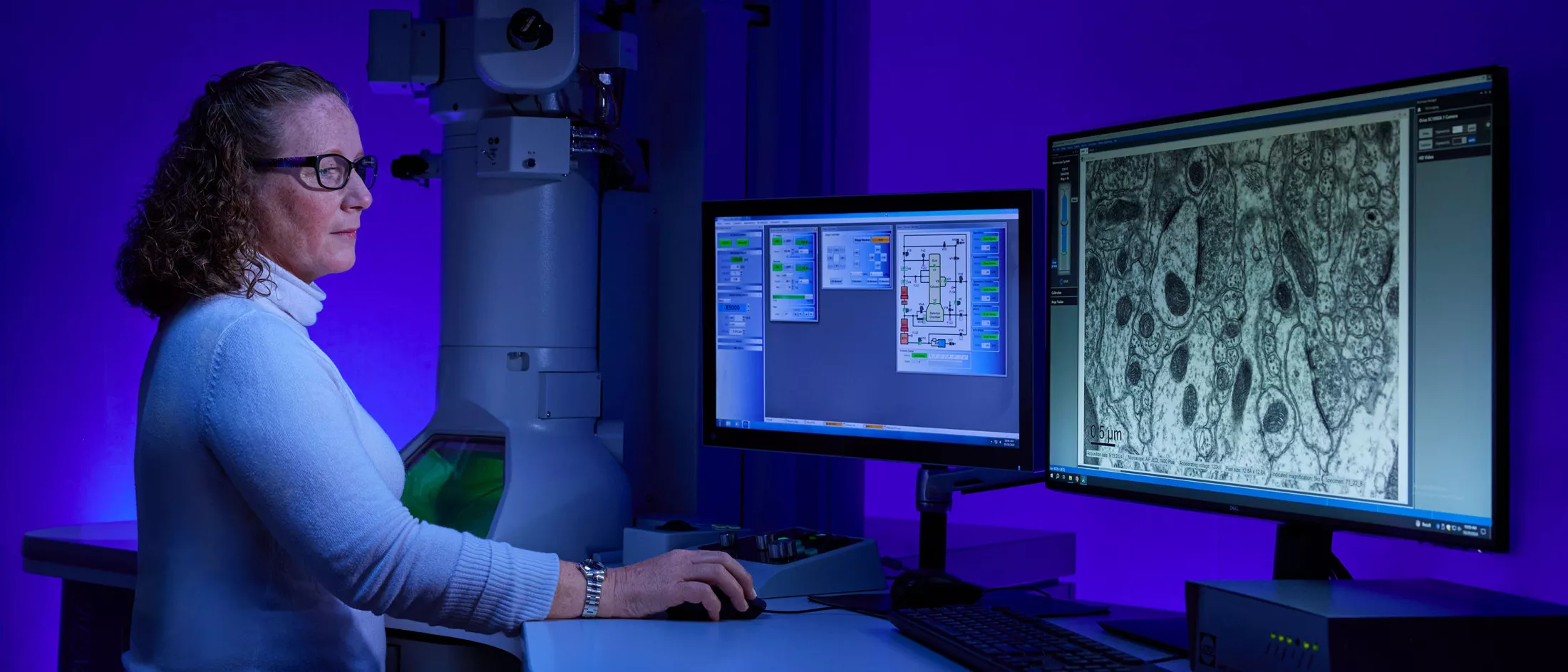